By Watershed Protection Program Director Lauren McGrath; Photos by Kate Etherington
On August 16, 2022, Executive Director Kate Etherington and the Watershed Team attended the Lenape Nation of Pennsylvania’s 5th Rising Nation River Journey and signed the Treaty of Renewed Friendship. The Lenape people are the original inhabitants of Delaware, New Jersey, Eastern Pennsylvania, and Southern New York, and were stewards in the Delaware River Watershed for over 10,000 years before the arrival of European settlers.
The group of nearly 50 individuals and organizations gathered in the sunny courtyard of Founders Hall at Haverford College, a longtime collaborator with the Lenape Nation, to celebrate the culmination of the River Journey. The ceremony began with smudging, or burning of ceremonial incense, performance of drumming and singing and the sharing of Lenape stories to share the significance of this event by Chief Shelley DePaul, Chief Gentle Moon Demund, and Tribal Council Storykeeper, Adam Waterbear DePaul. The Treaty signing then began, with supporting organizations and individuals taking turns to sign the document. “WCT recognizes the Lenape Nation as the original stewards of this land, and as an organization working to protect the land and waters within the Delaware River Watershed, we are honored to be invited to sign this Treaty, to carry on the tradition of land and water stewardship, and look forward to learning from and partnering more closely with the Lenape Nation in the years to come,” said Kate Etherington. The three week River Journey takes place every four years in August. The goal of this trip down the Delaware (or Lenape Sipu), is to “promote awareness that the Lenape people living in Pennsylvania are carrying on their ancestral traditions, culture and spiritual beliefs, and that they are engaged in numerous projects to provide practical ways for all citizens to respect and protect our homeland and the health, welfare, and future of the next seven generations of our children”1 as well as to elevate that Pennsylvania is one of the only states that does not recognize its indigenous peoples.
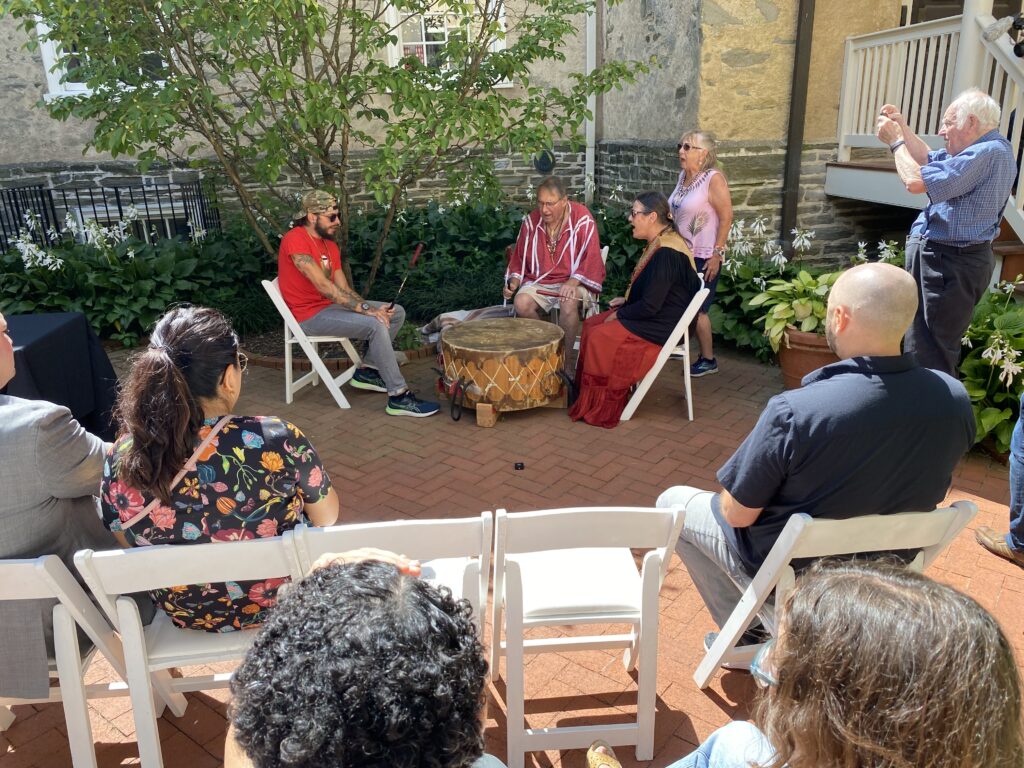
The Treaty of Renewed Friendship is an acknowledgement that the Lenape are the indigenous caretakers of these lands and signatories agree to support the Lenape Tribe in their own unique way including: Hosting Cultural / Educational programs, partnering as caretakers of the Lenape homeland and Delaware River, assisting in Lenape Language revival projects, assisting in displays/exhibits of Lenape culture, helping the Lenape people to obtain and/or protect sacred land sites, encouraging updated curriculum in public schools, attending Lenape functions, volunteer service and support, distributing information, financial assistance1. In signing the Treaty of Renewed Friendship, WCT joins a growing list of like minded organizations to collaborate and work towards shared goals of moving forward in our work of promoting respect and protecting the natural environment for future generations. Click here to read the full Treaty.
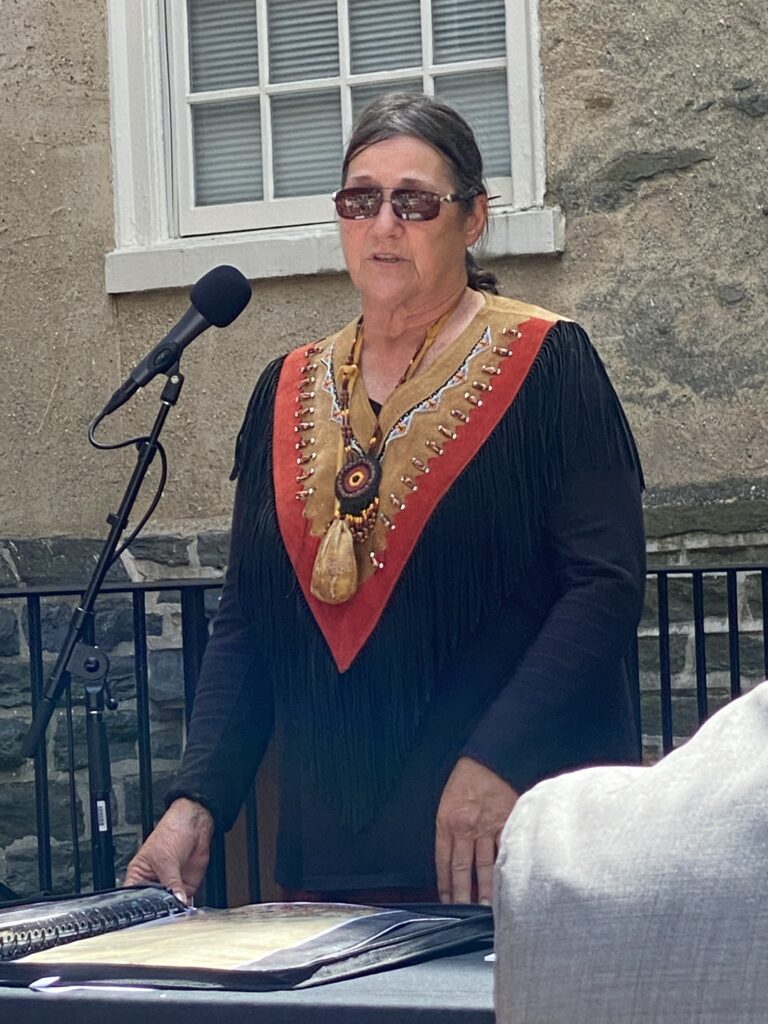
From a historical perspective, WCT’s program area contains at least one important landmark in Lenape Nation history. Okehocking Reservation (a portion of which is now known as Okehocking Preserve), is one of the first Reservations in the United States and many of the Lenape People were moved onto this parcel of land as colonization settled into the Southeastern Pennsylvania landscape. The majority of the Lenape in this Reservation were forcibly removed and driven westward to form communities in Oklahoma, Kansas, Ohio, Indiana, Wisconsin in the late 1800’s2.
In addition to the historic significance of the Lenape People in Chester County, there is a growing understanding in the scientific and land trust communities that to create climate resilient environments, we must turn back to the ancestral stewards of the landscape and work towards incorporating the knowledge and ethic of these cultures into the work of conservation. WCT is grateful for the opportunity to sign the Treaty of Renewed Friendship with the Lenape Nation of Pennsylvania and focus efforts in the next four years to elevate their voices and knowledge, grow in our understanding of stewarding the landscape, and building meaningful connections with the natural environment.
Click here to learn more about the Lenape Nation of Pennsylvania and to see upcoming events and educational opportunities (including language classes!) from the Lenape Nation, and be sure to visit the curated exhibit at Haverford College from January through July of 2023.
Wanishi!
References: